Healing Beams

Radiation Oncology has come a long way since its origins as an offshoot of the Department of Radiology and later as a program of the Department of Oncology. Although it didn’t receive departmental designation until 2003, the history of excellence in cancer research and patient care and the integral role it has played in the management of cancer have deep roots.
What started 50 years ago as a program built around the cancer-fighting power of targeted X-ray beams has evolved significantly. These healing rays are still essential to the care we provide, but since becoming a department 20 years ago, radiation oncology has grown into much more. Molecular radiation sciences, knifeless radiosurgery, proton beams, radio sensitizers, nanoparticles, radiolabeled drugs, targeted and immune-stimulating therapies, informatic systems, efficiency models, and inventions that propel research and make the clinic safer are now part of our 21st-century cancer science and medicine.
Building upon the strength and the work of early pioneers, our radiation oncology and molecular radiation sciences experts have earned recognition as world leaders in developing transformational concepts and translating basic developments into novel therapies that have changed the standard of care and improved the lives of people with cancer.
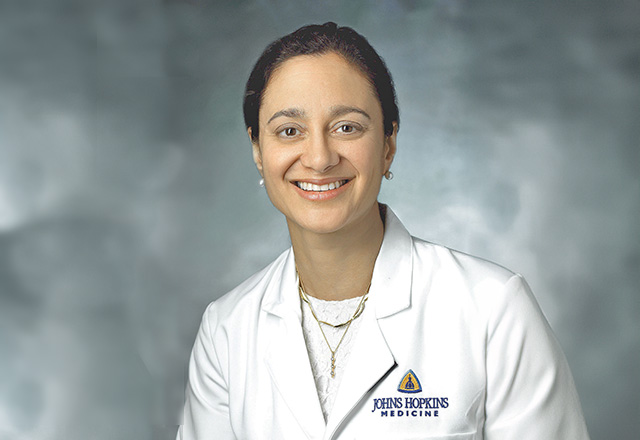
“Caring about our patients is our top priority,” says Akila Visvanathan, M.D., M.P.H., director of the Department of Radiation Oncology and Molecular Radiation Sciences. “Combining advanced technology with compassionate care is the hallmark of Johns Hopkins Medicine. Innovations beyond standard technology set us apart from a routine experience.”
Growing Pains
Radiation Oncology started with a small team working in the basement of the Halstead Building as they awaited the opening of the Cancer Center in 1977. Computer technology was limited, as were the machines that delivered radiation to patients.
Stanley Order, M.D., who was director of the Division of Radiation Oncology from 1975–1990, and Moody Wharam, M.D., director from 1990–2000, were the first two faculty members in radiation oncology. The existing radiation-delivering machinery had not caught up with the forward-thinking ideas of the Cancer Center’s early radiation oncology pioneers, and Order and Wharam worked together to get the equipment up to date. They converted two antiquated cobalts, treatment machines no longer in use today that produced a beam of gamma rays from a cobalt isotope, to five linear accelerators, which use targeted, high energy X-rays. They also added a cobalt unit and two simulators.
The Radiation Oncology Department at Johns Hopkins was among the first in the nation to break off from the Department of Radiology and Radiological Sciences to join forces with the Department of Oncology to tackle the cancer epidemic that had warranted a national war against cancer. Johns Hopkins was home to one of just a handful of strong academic programs in radiation oncology.
When the Center opened in 1977, it had all the latest technology and equipment available at the time. It also had more cancer patients than the physical space could accommodate. The radiation oncology clinic had to expand to twice its original size to accommodate the growing patient load.
Years later, there were two additional expansions, one with the opening of the Kimmel Cancer Center’s Harry and Jeanette Weinberg building and another with the satellite facility at Green Spring Station. Additional facilities were again added at Suburban Hospital in Montgomery County and Sibley Memorial Hospital in Washington, D.C., which would later become home to the proton therapy center.
Pioneering Discoveries

Strong leadership has helped pave the way for pioneering discoveries. Order developed radiolabeled antibodies to treat liver cancer. He was also ahead of his time, developing a version of telemedicine. In 1989, the Cancer Center established collaborative radiation oncology services with St. Agnes Hospital in Baltimore and Chambersburg Hospital in Pennsylvania.
Patient information from Chambersburg, such as X-rays and charts, was relayed to the Cancer Center via computer, fax and video telephone hook-up. A telephone conference brought physicians from all of the hospitals together for case review.
Wharam, who specialized in pediatric oncology, was also a pioneer. When our Center opened, just 50% of children diagnosed with cancer survived. The National Cancer Institute appointed four study groups to investigate common childhood cancers, and Wharam received the unusual distinction of being named to two of these groups. From 1980 to 1990, he served as director of the radiation oncology committee of the pediatric oncology group, a U.S. and Canadian collaborative group that studied childhood cancers. His roles in these premier groups made him an active participant in all of the pivotal pediatric cancer research at the time. It was research that led to dramatic increases in pediatric cancer survival rates. The four separate groups have since merged into one, known as the Children’s Oncology Group.
Wharam, who died in 2018, was part of another first, when he collaborated with pediatric oncologist Bridget Leventhal in a ground-breaking 1980s study of treatment reduction in Hodgkin’s lymphoma to prevent harmful toxicities. Their research led to refinements in therapy that allowed certain patients to receive less radiation or forgo it altogether without an increased risk of recurrence.
He also developed the standard of care for rhabdomyosarcoma, a childhood cancer of the connective tissue that attaches muscles to bones, combining chemotherapy and radiation therapy, a treatment so effective it remains the standard today.

In the early 1990s, years before advanced radiosurgery equipment had been developed, he innovated a way to deliver radiation very precisely to preserve vision for a toddler diagnosed with cancer in both eyes.
Wharam’s pioneering influence earned the department distinction as one of just a select few in the nation with expertise in treating pediatric patients, and this was instrumental in helping the department gain approval for a proton therapy center, which includes a specialized pediatric team.
“Our program grew into the best one in the country. We have first-class scientists and clinicians and the finest physicists, residents, nurses, radiation therapists and dosimetrist in the business,” said Wharam.
Theodore DeWeese, M.D., now interim dean of the Johns Hopkins University School of Medicine, was named the first director of the Department of Radiation Oncology and Molecular Radiation Sciences.
DeWeese, a prostate cancer expert, collaborated with researcher Shawn Lupold, Ph.D., to develop an aptamer, small molecules that work like antibodies to target unwanted things in our bodies, like cancer. The aptamers deliver silencing RNAs specifically into cancer cells to render cancer cells vulnerable to the DNA damage caused by radiation therapy while protecting normal cells.

DeWeese was instrumental in bringing proton therapy to the Kimmel Cancer Center and advancing radiation oncology research, recruiting Marikki Laiho to head molecular radiation sciences and inventor John Wong as chief physicist.
An Inventor
“Radiation oncology requires the right balance of technology development, laboratory research and dissemination of knowledge for clinical decisions,” said Wong, now retired.
He was the inventor of many key instruments used in radiation oncology treatment and cancer research.
Among his inventions is the ABC interactive device, which coordinates breathing with radiation treatment. As patients breathe, tumors move, and ABC locks the breath in place for short, comfortable periods to make sure the radiation hits its cancer target.
ABC has been enhanced with 4D CT and MRI imaging. Radiation oncologist and lung and esophageal cancer expert Russell Hales, M.D., explains that the fourth dimension is the capture of movement. The 4D imaging captures a few seconds of a patient’s breathing, and these data are used to predict how and where the tumor will move throughout treatment.
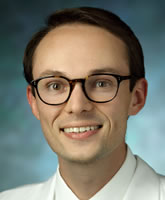
Hales is conducting critical research to validate the ability of image-based technologies to predict tumor movement during radiation treatment.
“We are one of the few places that are doing this kind of research,” says Hales. When completed, he says the research should produce the most accurate measurement of tumor movement.
Wong was also the inventor of cone-beam computed tomography, which has become an integral part of radiation oncology treatment and research. CT imaging delivers clear images of bone, soft tissue and tumor, making it a desirable guidance system for radiation treatment.
The Raven quality assurance device, which connects to machines and quickly performs a series of measurements to ensure they are functioning correctly, and the CT couch, which integrates image guidance with treatment delivery, are also among Wong’s inventions.
Another one, called the Small Animal Radiation Research Platform (SARRP), gave researchers the ability to study human therapies in animal models before they are taken to the clinic, and was essential to developing more effective and safer therapies. SARRP is a downsized version of a human machine, allowing researchers to perform human-quality radiation delivery in animal models.
“In radiation oncology, we don’t have the means to study mechanisms in a living animal subject, and this machine helps us do that,” said Wong.
Molecular Radiation Sciences
“When we think about radiation therapy, it is high technology, but the complexity of cancer requires that we have a better understanding of the biology,” says Marikki Laiho, M.D., Ph.D., director of molecular radiation sciences and the Willard and Lillian Hackerman Professor of Radiation Oncology.
The work of Laiho and molecular radiation sciences researchers is aimed at better understanding the mechanisms cancer cells use to survive radiation therapies. They are looking for kinks in cancer cells’ armor that can be exploited to prevent them from sensing and repairing the DNA damage caused by radiation therapy.
“DNA damage is not unique, but our research focus is,” says Laiho. “There is not a radiation and molecular radiation sciences research program anywhere with the depth and focus we have here.”
Among the research projects are studies of cell repair molecules known as PARPs. Drugs called PARP inhibitors can sensitize cancers to radiation therapy and stop cancer cells from making repairs after therapy.
Understanding the signaling pathways involved in DNA damage — in particular the role they play in deadly glioblastoma brain cancer — is another area of study. Researchers are investigating the relationship between DNA and its marking by epigenetic tags and how they contribute to cancer cell development and survival. Although each has been studied individually, molecular radiation sciences researchers were among the first to explore how they work together in cancer.
Researchers also want to figure out why some tumors respond to radiation therapies and chemotherapies while others do not. Using genetic tools created to selectively introduce cancer mutations into tumor cells, researchers can explore and observe how the cells respond to cancer therapies and devise strategies to combat treatment resistance.
Heat increases the sensitivity of cancers cells to radiation therapy, and another approach uses magnetic nanoparticles that generate heat when exposed to magnetic fields. Sensitizing cancer cells with heat could make it possible to lower radiation doses or treat cancers that would be otherwise unresponsive.
Testosterone, a hormone that prostate cancer cells need to survive, can also play a role in their demise. Researchers believe testosterone causes breaks in the DNA that could make cancer cells more vulnerable to treatment with radiation therapy. Short pulses of testosterone — enough to stimulate the brakes but not too much to stimulate the cancer — followed by radiation therapy to cause even more DNA breaks, can overwhelm and kill prostate cancers.
A biodegradable hydrogel with the consistency of toothpaste could help protect vital organs from damage during radiation therapy. The hydrogel separates the tumor from organs by about a finger’s width, and this extra space allows the radiation to fall off and protects surrounding tissue and organs. Hydrogel spacers are most commonly used in the treatment of prostate cancer, but they are being studied in pancreatic cancer, liver cancer, gynecologic cancers, and head and neck cancers.
Laiho’s own research project is focused on a cellular machinery called POL1, which cancer needs to survive. POL1, she says, is necessary for cancer cells to maintain their rapid growth. In her laboratory studies using human cells, new small-molecule inhibitors break down this critical activity. The next step is clinical trials.
Oncospace
Radiation Oncology researchers led the way in applying technology to precision medicine — treatments tailored to the unique aspects of each patient’s cancer.
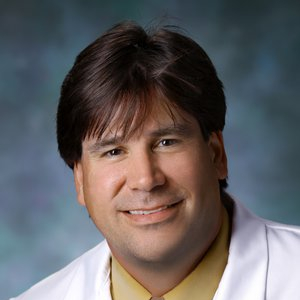
Physicist Todd McNutt developed a computerized data mining system called Oncospace that analyzes data from prior patients who received radiation therapy to improve the treatment of new patients. It sorts through all of the data on patients with cancer treated at the Kimmel Cancer Center, taking into account and connecting all of the variables: age, underlying health conditions and other treatments patients are receiving, and figures out how all of these variables relate and influence toxicity and response to treatment. It evaluates the therapies that worked best for a particular cancer — as well as those that resulted in less than favorable outcomes — and generates an optimal treatment plan.
“The practice of cancer medicine naturally creates data,” says McNutt, “but for the first time in history, we have the technology to sift and sort through this data in completely new ways.”
The success of precise, individualized medicine, including the ability to determine which patients will benefit from a particular drug or treatment and which ones will not, rested on the ability to conquer big data. Oncospace was one of the first practical demonstrations of this promise.
“Todd has proven that large data warehouses of patient information collected from previously treated patients can be used to individualize treatment decisions for new patients,” said Ted DeWeese, interim dean of the Johns Hopkins University School of Medicine and former director of the Department of Radiation Oncology and Molecular Radiation Sciences.
Oncospace does more than collect and store data. It takes informatics to the critical next level with the capability to perform interactive analysis that informs clinical decision-making. Radiation oncologist and head and neck cancer expert Harry Quon provided the crucial link to put the system to test in the clinical practice.
“I could tell you very accurately where the radiation dose goes,” says McNutt, “The important question in treating patients is where should it go and where shouldn’t it go.”
Patients with head and neck cancer were the ideal choice for testing Oncospace in the clinical setting because they are among the most difficult cancers for radiation physicists and oncologists to plan. They often require 20 treatment revisions as the experts work to design a treatment plan that hits the cancer without causing damage to vital organs and glands, such as the voice box and salivary glands.
“We can build predictive models of toxicities and other side effects based on data we have collected from prior patients, including indicators that a patient may be at higher risk for certain treatment toxicities, and use this information to adjust the treatment plan,” explains McNutt.
More recently, they have begun incorporating data on disease response: Is the cancer stable? Has it progressed? Did it recur? Imaging used for treatment simulation is also being incorporated into the collected data to track the history of a tumor throughout treatment. It could help tell early on in the treatment of a cancer if a tumor is responding or if a change to the treatment plan may be necessary.
McNutt says this latest variation is the radiation oncology version of the work being done in cancer genetics and molecular biology using genetic biomarkers to track and monitor the response of cancers to drug therapies.
“It’s real-time, in-treatment monitoring” says McNutt. “The same way we use the system to relate the dose of radiation to the salivary gland to the loss of gland function, we can use it to relate treatment plans to treatment responses.”
The success in head and neck cancer has led to Oncospace now being applied to a variety of cancers, including lung, pancreatic and prostate cancers.
Radiosurgery
Stereotactic body radiosurgery (SBRT) is an option for some patients who have tumors that cannot be removed through traditional surgery. This treatment, sometimes referred to as knifeless surgery, uses high but super-focused doses of radiation to destroy tumors while also limiting side effects to nearby normal organs and tissue.
When pancreatic cancer SBRT is performed, tiny gold seeds, about the size of a grain of rice, are inserted by needle into the pancreatic tumor. They serve as reference points to help guide the radiation oncologist as they treat the cancer. Using the same needle that places the seeds, radiation oncologists remove tiny pieces of the tumor for genetic sequencing.
This unique research study proved that tumors could be sequenced through this small sampling of cells, and the genetic information could be used to individualize treatment in patients with inoperable pancreatic cancer.
“We were the first ones to try this, and we proved it could be done,” said Joseph Herman, M.D., former radiation oncology faculty member who led the initial study.
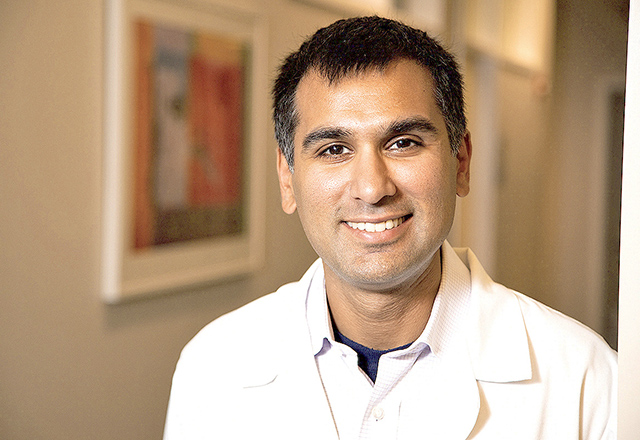
Stereotactic radiosurgery is also being used by Amol Narang, M.D., to get more people with pancreatic cancer to surgery. Building upon earlier research, he is using SBRT in pancreatic tumors that have attached to nearby blood vessels, making surgical removal of the entire tumor difficult or impossible.
Combining chemotherapy with radiosurgery in these cases helps shrink tumors away from the blood vessels, making surgical removal of the tumor possible for more patients. The immune-priming ability of radiosurgery is also being studied in clinical trials of people with pancreatic cancer.
The prevailing opinion in cancer research was that chemotherapy and radiation therapy suppressed the immune system, but Kimmel Cancer radiation oncologists proved that may not be the case.
Focused radiation, like what is used in stereotactic radiosurgery, they found, may actually stimulate an immune response. Collaborating with Kimmel Cancer Center immunology experts, radiation oncology experts used the small-animal radiation research platform to study a combination of radiosurgery and an immune therapy drug in animal models.
Radiation therapy is considered a localized treatment, but when combined with immune therapy, it gains an added systemic activity. Immune therapy prevents cancer cells from deploying immune-dampening regulatory cells. With the regulatory cells taken care of, radiation oncologists could use radiosurgery to enlist the entire complement of immune cells to fight the cancer: killer T cells, which, as the name implies, kill cancer cells; memory T cells, which remember the tumor cells and have the power to keep the cancer in check indefinitely; and B cells, which generate antitumor antibodies that interfere with the cancer cells’ DNA and stop them from replicating new copies.
This initial study was led by former radiation oncology resident Andrew Sharabi, M.D., Ph.D., and former Bloomberg~Kimmel Institute for Cancer Immunotherapy researcher Charles Drake, M.D., Ph.D. Sharabi’s research was selected from thousands of submissions as a featured presentation at the 2014 annual meeting of the American Society for Radiation Oncology. It was the first basic science research to be highlighted at the meeting in over a decade.
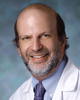
For brain tumor expert Lawrence Kleinberg, M.D., SBRT means he can safely treat the spread of cancer in the brain.
“Before we had radiosurgery, we had to treat the entire brain with radiation and that, of course, caused many side effects,” said Kleinberg. “Now, in most situations, we can treat very small areas of the brain with almost no side effects.”
As a result, the Kimmel Cancer Center radiosurgery team offers one of the few treatment options for patients with spread of cancer to the brain.
Brachytherapy
Brachytherapy, a widely used and promising tool of radiation therapy, is commonly used in the treatment of prostate cancer and gynecologic cancers as an alternative to surgery.
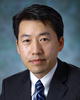
In prostate cancer, radiation is delivered to the prostate via tiny seeds about the size of a grain of rice. Accurate placement of these seeds has been the biggest challenge, but brachytherapy expert Danny Song, M.D., has been a leader in pioneering guidance systems that ensure the seeds are deployed correctly.
To destroy prostate cancer, about 50–100 seeds are placed by needle in the prostate while the patient is under general anesthesia. The greatest limitation to brachytherapy, Song says, was that there was no good, real-time way to see if the seeds were getting to the correct place. X-ray showed the seeds but did not provide a clear image of the prostate, and ultrasound shows the prostate well but not the seeds.
He decided to combine the two technologies into one. In a collaboration with Johns Hopkins University engineers, Song developed RadVision. As seeds are placed, multiple X-rays are taken and fed into a computer to generate a 3D arrangement of seeds. The seed positions are then superimposed over ultrasound images to ensure the right number of seeds has been placed and to guide the placement of additional seeds, if needed.
RadVision received FDA approval after a clinical study that proved it provided the most accurate seed placement. Patients who have received brachytherapy often also require external beam radiation to compensate for an inadequate seed placement, but Song says seed placement with RadVision is so accurate it may eliminate the need for additional radiation treatments.
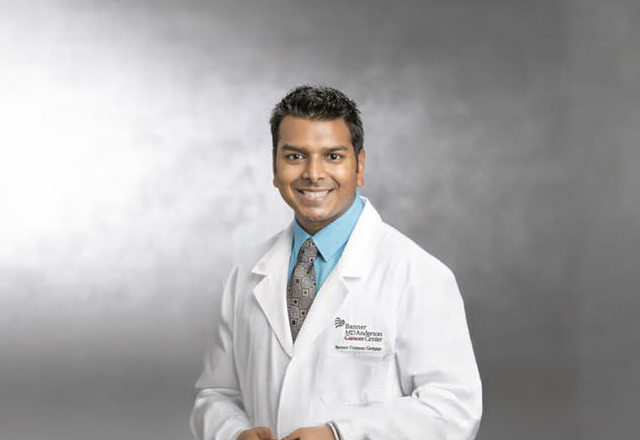
In 2023, a high-dose rate prostate brachytherapy program was launched at the Kimmel Cancer Center at Sibley Memorial Hospital under the direction of Rachit Kumar, M.D.
Viswanathan was the first in the U.S. to use real-time magnetic resonance (MR)-guided interstitial brachytherapy for the treatment of gynecologic cancers.
Using active MRI guidance, physicians insert several hollow catheters into the tumor. Tiny radioactive seeds, tethered together by a long thread, are inserted into the catheters and remain there for about 10 minutes, providing a rapid but high dose of radiation to control cancer cells. A computer manages the insertion and removal of the seeds, ensuring a precise dose throughout the tumor.
“Gynecologic cancers can grow very fast, and require a very focused, high dose to attack the tumor,” Visvanathan explains.
The entire outpatient procedure takes just a few hours, and provides a lifetime of benefits to patients.
“I wanted women who have inoperable cancer that is limited to the gynecologic area to be cured of this cancer,” she says.
Women who have surgery for cancer often lose their entire gynecologic tract, including the cervix, she explains. Brachytherapy preserves these organs, which is particularly important for young women.
The type of MR technology she uses is unique. Working with the team of physicists who write special codes to direct the MRI scanner, she can look inside a tumor and provide a variety of details not available with traditional MRI. Outcomes are excellent for cervical cancer and recurrent uterine, vulvar and vaginal cancers, Visvanathan says, with published data showing MR-guided brachytherapy survival rates of over 90%. As a result, Visvanathan is in demand, seeing about 300 patients a year from all over the world. She is training other radiation oncologists to perform the procedure, which she recently expanded to the Kimmel Cancer Center at Sibley Memorial Hospital.
Theranostics
A new approach, dubbed theranostics because it combines the diagnostic properties of molecular imaging with cancer therapy, was developed at the Kimmel Cancer Center.
Led by imaging experts Martin Pomper, M.D., Ph.D., and Zaver Bhujwalla, Ph.D., and radiation oncologist Ana Kiess, M.D., Ph.D., the novel approach takes advantage of important molecular components of cancer and allows researchers and clinicians to see inside the cancer cells and view them as they are being treated.
The team developed ultra-tiny structures called nanoparticles filled with a drug that kills cancer cells and sensitizes them to radiation and a radiopharmaceutical or cell imaging agent. The nanoparticle is targeted to PSMA, which is present at high levels in prostate cancer, so that it zeroes in on and delivers its anticancer payload specifically to prostate tumors. The particle is labeled with a radioactive isotope, which can be imaged or used to treat cancer. It is given intravenously so that it can attack cells growing anywhere in the body.
Kiess and Pomper worked with chemists to modify a drug called LU-PSMA-12 to make it specific to cancer cells and less likely to go into normal cells. The drug is being studied in multicenter clinical trials of advanced prostate cancer.
Less Is More
The ability to shorten the several-week course of radiation therapy is a new area of research radiation oncologist and colorectal cancer specialist Jeffrey Meyer, M.D., is studying — whether to abbreviate a typical five-week course of radiation therapy to five days, followed by chemotherapy two months later and then surgery. The regimen is easier for patients, with fewer interruptions to their normal routines.
In early studies, long-term outcomes appear to be as good as the traditional, longer courses of radiation therapy, Meyer says, but he adds that the research continues.
Jean Wright, M.D., director of the Breast Cancer Program’s radiation oncology service, is studying a similar approach for breast cancer.
In the 2000s, radiation therapy was delivered over five to seven weeks for almost all patients needing breast radiation, Wright says. About 15 years ago, studies found that shorter, three to four-week courses were as effective for patients at lower risk for breast cancer recurrence, and, she says, they have moved to these shorter courses for many patients.
She is also exploring the benefits of an even shorter, one-week, high-dose, whole breast radiation approach. The very convenient course has to be weighted for each patient, depending on their breast cancer risk as well as personal preferences and priorities, Wright says.
“This is another great example of how our field is evolving toward patient-centric, tailored treatments,” says Wright.
Proton Therapy
Proton therapy uses charged particles, rather than photon therapy’s high-powered X-rays beams, to kill cancer. Proton does not replace photon radiation therapy, but it is another important tool our experts have to treat cancer.
The Johns Hopkins Proton Therapy Center opened in 2019 at Johns Hopkins’ Sibley Memorial Hospital. It is one of only about 40 centers in the U.S., and one of a few with dedicated proton beams for research and a specialized pediatric team.
One of the most comprehensive in the world, the Proton Therapy Center has technology to deliver the most advanced and patient-centered care. It combines cancer treatment excellence across all disciplines with proton therapy excellence, building upon a lengthy history and strong foundation of pioneering discoveries in radiation therapy.